The ultra-fast cancer treatments which could replace conventional radiotherapy
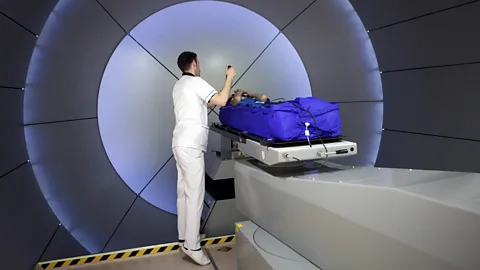
A pioneering new treatment promises to tackle a wider range of cancers, with fewer side-effects than conventional radiotherapy. It also takes less than a second.
In a series of vast underground caverns on the outskirts of Geneva, Switzerland, experiments are taking place which may one day lead to new generation of radiotherapy machines. The hope is that these devices could make it possible to cure complex brain tumours, eliminate cancers that have metastasised to distant organs, and generally limit the toll which cancer treatment exerts on the human body.
The home of these experiments is the European Laboratory for Particle Physics (Cern), best known to the world as the particle physics hub that developed the Large Hadron Collider, a 27 kilometre (16.7 mile)-long ring of superconducting magnets capable of accelerating particles to near the speed of light.
Arguably Cern's crowning achievement was the 2012 discovery of the Higgs boson, the so-called "God Particle" which gives other particles their mass and in doing so lays the foundation for everything that exists in the universe. But in recent years, the centre's unique expertise in accelerating high-energy particles has found a new niche – the world of cancer radiotherapy.
Eleven years ago, Marie-Catherine Vozenin, a radiobiologist now working at Geneva University Hospitals (Hug), and others published a paper outlining a paradigm-shifting approach to traditional radiotherapy treatment which they called Flash. By delivering radiation at ultra-high dose rates, with exposures of less than a second, they showed that it was possible to destroy tumours in rodents while sparing healthy tissue.
Its impact was immediate. International experts described it as a seminal breakthrough, and it galvanised fellow radiobiologists around the world to conduct their own experiments using the Flash approach to treat a wide variety of tumours in rodents, household pets, and now humans.
The Flash concept resonated as it addressed some of the long-standing limitations of radiotherapy, one of the most common cancer therapies, which two-thirds of all cancer patients will receive at some point in their treatment journey. Typically delivered through administering a beam of X-rays or other particles over the course of two to five minutes, the total dose is usually spread across dozens of individual treatment sessions over up to eight weeks, to make it more tolerable for the patient.
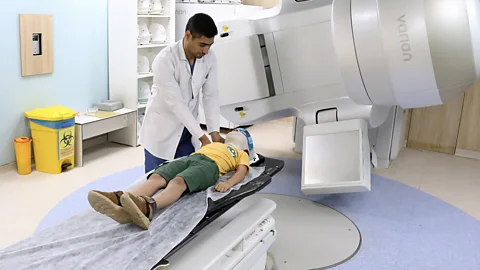
Over the past three decades, advanced imaging scans and state-of-the-art radiotherapy machines have made it possible to target an individual tumour with increasing precision. But the risk of damaging or deadly side effects is still present.
Vozenin cites the example of paediatric brain tumours, which can often be cured by blasting the brain with radiotherapy, but at a great cost. "The survivors are often left with lifelong anxiety and depression, while the impact of the radiation affects brain development, causing significant loss of IQ," she says. "We're [sometimes] able to cure these kids but the price they pay is high."
Billy Loo, a professor of radiation oncology who runs the Flash sciences lab at Stanford University School of Medicine in the US, explains that tumours, especially those of larger volume, are rarely neatly segregated from the surrounding tissue. This means it's often next to impossible to avoid harming healthy cells, so oncologists are often unable to use as high a dose as they would like, says Loo.
Cancer specialists have long believed that being able to boost the radiation dose would greatly enhance their ability to cure patients with difficult-to-treat cancers, according to Vozenin. For example, research has previously indicated that being able to increase the radiation dose in lung cancer patients with tumours that have metastasised to the brain could improve survival.
In recent years, animal studies have repeatedly shown that Flash makes it possible to markedly increase the amount of radiation delivered to the body while minimising the impact that it has on surrounding healthy tissue. In one experiment, healthy lab mice which were given two rounds of radiation via Flash did not develop the typical side effects which would be expected during the second round. In another study, animals treated with Flash for head and neck cancers experienced fewer side effects, such as reduced saliva production or difficulty swallowing.
Loo is cautiously optimistic that going forwards, such benefits may also translate to human patients. "Flash produces less normal tissue injury than conventional irradiation, without compromising anti-tumour efficacy – which could be game-changing," he says. An additional hope is that this could then reduce the risk of secondary cancers, resulting from radiation-induced damage later in life, although it is still too early to know if that will be the case.
Now, increasing numbers of human trials are beginning to take place around the world. Cincinnati Children's Hospital in Ohio, US, is planning an early stage trial in children with metastatic cancer that has spread to their chest bones. Meanwhile, oncologists at Lausanne University Hospital in Switzerland are conducting a Phase 2 trial – where the details are finessed, including the optimum dose, how effective the treatment is and if there are any side effects – for patients with localised skin cancer.
But the next phase of research is not only about testing whether Flash works in people. It's also about identifying which kind of radiation is the best one to use.
A choice of particles
From carbon ions to protons and electrons, there are many ways of delivering radiotherapy, each with different applications and challenges. One of the most precise forms of radiotherapy is hadron therapy, delivered with carbon ions. But there are only 14 facilities which can deliver this in the entire world, each one costing an estimated $150m (£122m). Currently this therapy is delivered using a conventional dosing regime, in which the radiation is delivered over several minutes. However, with the Flash protocol the ions would be delivered in less than a second.
"High energy electrons can be used to treat superficial tumours in the skin," says André-Dante Durham Faivre, a radiation oncologist at Hug. "Photons, i.e. X-rays, or protons [a type of subatomic particle], can be used to treat deeper tumours, while we save carbon ions and helium particles for very specialised cases, as it's only very, very big clinical centres that can offer that type of treatment. The particle accelerator needed to administer carbon ion radiotherapy is the size of a building."
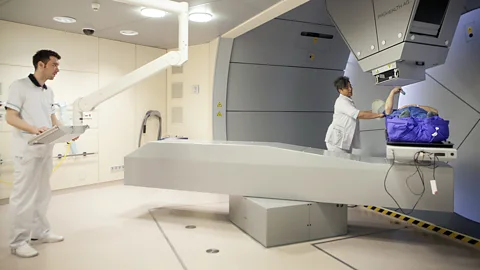
This is one tricky problem with Flash therapies. Because creating subatomic particles requires extremely complex particle accelerators, at the moment this treatment can only be delivered via vast pieces of equipment in specialist centres, which is expensive. This means patients will most likely need to travel long distances for their treatment – and while researchers hope that eventually Flash will be available to everyone who needs it, at the moment treatments such as proton therapy are only available to a relatively small minority of patients.
So far, protons have been the particle of choice for human Flash trials, both because they can penetrate up to 30cm (12in) into the body, enabling them to reach relatively deep internal organs, and because existing proton radiotherapy machines can be adapted relatively easily to deliver Flash dose rates.
In 2020, the University of Cincinnati Medical Centre launched the first ever clinical trial of Flash proton radiotherapy in patients whose primary cancer had metastasised to the bones, with early results suggesting that the treatment was just as effective as conventional radiotherapy and the incidence of adverse events was similar. Now, radiation oncologists at the University of Pennsylvania Perelman School of Medicine are hoping to launch their own trial later this year in patients with recurrent head and neck cancer.
"These are patients who have few other options as their tumours are impossible to remove via surgery," says Alexander Lin, professor of radiation oncology at the University of Pennsylvania, who will lead the proposed trial. "Going through another course of standard radiotherapy would potentially lead to dangerous side effects such as jaw fractures, mouth wounds and even potentially fatal damage to the carotid artery. We believe that proton Flash will be less toxic."
A practical challenge
However, if proton Flash were to be approved by regulators in future, Durham Faivre says that one of the disadvantages is that the machines required are still relatively large, meaning the treatment could only be administered in a select number of centres, restricting patient access.
Now Cern are working with researchers at Lausanne University Hospital and the French company TheryQ to try and develop a new form of accelerator which delivers even more radiation – described as very high energy electrons – at Flash dose rates. And according to Durham Faivre, Hug researchers are currently in discussions with commercial partners to develop an X-ray Flash machine.
Such accelerators could enable the benefits of Flash to be applied to deep tumours without requiring a vast machine, says Durham Faivre. The ultimate goal is to make it possible for any hospital with radiotherapy equipment to be able to provide Flash. "We believe that X-ray Flash machines could in time replace existing conventional X-ray machines," he says.
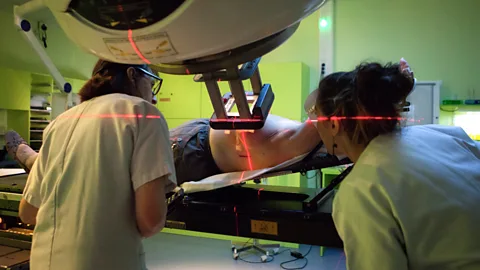
In particular, Durham Faivre is optimistic that newer accelerators could allow oncologists to tackle more complex tumours such as glioblastoma, the most common form of brain cancer and one of the deadliest forms of the disease, with a five-year survival rate of just 5%.
Following on from the University of Cincinnati trial, oncologists are also hopeful that Flash machines could improve the treatment of various forms of metastatic disease (where the cancer has spread from its primary location) and actually cure patients who were previously considered incurable. Loo predicts that Flash could be used to destroy the primary and secondary tumours, then followed by chemotherapy or immunotherapy to eliminate the microscopic cancer cells which are enabling the disease to spread.
"Metastatic cancers involve large volumes of the body because of their diffuse distribution," says Durham Faivre. He explains that this means they're usually hard to cure, because it wouldn’t be possible to deliver enough radiation to the body’s tissues to kill all the cancerous cells. If you did, the patient may not survive the effects of the radiation on previously healthy tissue. But newer treatments are changing this, he says, particularly in people with limited metastases. “Flash offers the prospect of safely treating many more metastases," he says.
Another hope is that Flash could ultimately help make radiotherapy more accessible to all.
The radiotherapy gap
At last September's UICC World Cancer Congress – a conference that brings together cancer experts from around the world – Katy Graef, vice-president of the non-profit Bio Ventures for Global Health, highlighted a major challenge in global health which is sometimes referred to as "the radiotherapy gap".
Using data compiled by the Lancet Oncology Commission, Graef described how there are only 195 radiotherapy machines in the whole of sub-Saharan Africa, compared with 4,172 in the US and Canada. With the annual incidence and mortality from cancer expected to double across the African continent by 2040, she explained that it has been projected that the region will require more than 5,000 additional machines in the next two decades, a demand which many nations will struggle to afford.
In December, a new review of national cancer control plans around the globe highlighted how the radiotherapy gap extends beyond Africa to many low and middle-income countries. "Only about 10% of cancer patients in low-income countries have access to radiotherapy, compared to 90% in high-income countries," says Lisa Stevens, director of the programme of action for cancer therapy at the International Atomic Energy Agency, and one of the authors on the paper. "The integration of radiotherapy into cancer control strategies is more crucial than ever."
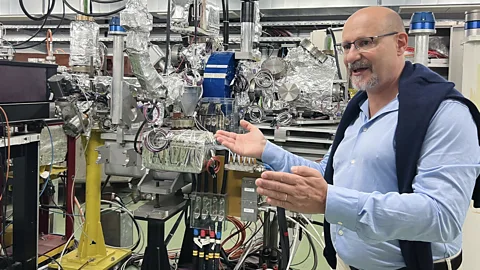
The challenges behind these statistics go beyond the mere costs of the machines. In hot and humid environments, radiotherapy particle accelerators often break down, and with few trained technicians, repairs can take time. As a result, the International Cancer Expert Corps (ICEC) have launched an initiative called Project Stella, in partnership with Cern and several UK universities, which aims to develop next-generation accelerators with integrated software that can predict faults in advance and streamline maintenance, enabling countries to make the best use of the machines they have by minimising downtime.
But Durham Faivre is optimistic that Flash machines could also have a role to play, eventually making it easier for cancer patients living in low- and middle-income countries to receive the treatment they need. Instead of needing to repeatedly travel long distances over the course of many days and weeks to receive multiple radiotherapy sessions, Flash could enable them to receive it all in either a single session or a small handful of sessions. Because each treatment takes less than a second, it would also enable doctors to treat many more patients in a single day.
"If we can get a normal sized machine that fits in all the hospital bunkers in the world and can administer Flash, that can allow countries to treat many more patients," says Durham Faivre. "If instead of treating 50 patients a day, you can treat 150, you're massively increasing your capacity, and your ability to handle the public health need."
Many experts feel that this would also yield substantial cost savings benefits for high-income countries, as well as having a potentially enormous improvement for patients' quality of life.
"It should be a more cost-effective treatment once the initial investment is made, since much fewer treatments are needed," says Constantinos Koumenis, professor of radiation oncology at the University of Pennsylvania Perelman School of Medicine in the US. Savings to the health system could also come due to fewer hospitalisations due to complications, he adds.
The first step, Koumenis explains, is investigating just how good Flash is – and whether it’s actually better than standard radiotherapy.
--
For trusted insights into better health and wellbeing rooted in science, sign up to the Health Fix newsletter, while The Essential List delivers a handpicked selection of features and insights.